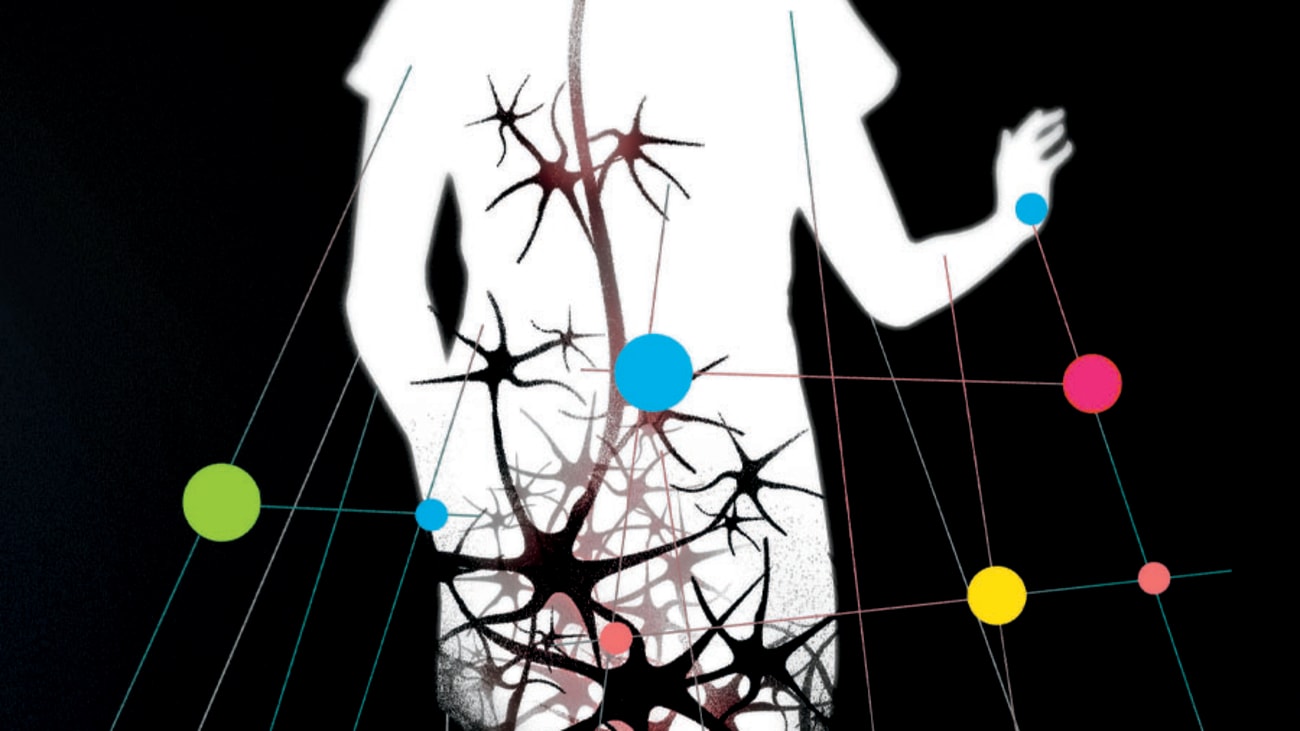
A first-of-its-kind stem cell therapy for ALS passes a critical safety benchmark, advancing the search to slow down, reverse and prevent the disease. In a parallel study, investigators are growing patient-derived stem cells to model ALS, hoping to uncover its mechanisms and classify it with more specificity. Can the cure to this degenerative condition lie in the endlessly regenerative power of stem cells?
First, her hands stiffened. Then she developed a limp. Ashley Fisher was 48 when she was diagnosed with amyotrophic lateral sclerosis (ALS). During the following three years, as the unstoppable disease took hold of her body, she lost the ability to work, hike by the beach or shop swap meets on Saturday mornings. So, she sought out what she could do with the time she had left: She enrolled in a clinical trial.
Even after paralysis stole her speech, Ashley consented to a five-hour spine operation, took immunosuppressive drugs for a year and underwent extensive testing at Cedars-Sinai’s ALS Clinic, where a team monitored the impact of the procedure on her body—specifically, one of her legs. Their goal: to test the safety of a combination stem cell/gene therapy to treat the rare neurodegenerative disease, caused by the unexplained, unstoppable death of motor neurons in the brain and spinal cord.
Moments after Ashley’s death, in May 2021 at a hospital in Oregon, her daughter, Courtney Fisher Olsen, relayed to a nurse the urgent instructions impressed upon her in the previous months: Call Cedars-Sinai and ask them to collect Ashley’s spinal tissue.
“She made this research a priority, and she was really proud of it,” Courtney says. “She would do anything to be part of finding answers.”
Ashley, along with the 17 others in the study, gave investigators their only opportunity to make a critical advance: The trial proved, for the first time, the safety of the implantation into the lumbar spinal cord of specialized stem cells—neural progenitors—engineered to express a powerful growth factor known to protect neurons. The findings, published in September 2022 in Nature Medicine, cleared investigators to study the therapy’s efficacy and continue refining the approach they hope that, ultimately, will slow or stop the disease.
"These patients are the heroes of this research. They knew we weren’t going to cure their disease, only pursue whether the cells and this surgical approach were safe. We are encouraged enough by this approach to proceed in an attempt to slow disease progression.”
Richard Lewis, MD
“These patients are the heroes of this research,” says Richard Lewis, MD, director of the Electromyography Lab and principal investigator of the study at the ALS Clinic. “They knew we weren’t going to cure their disease, only pursue whether the cells and this surgical approach were safe. We are encouraged enough with the results to proceed to more patients and attempt to slow disease progression.”
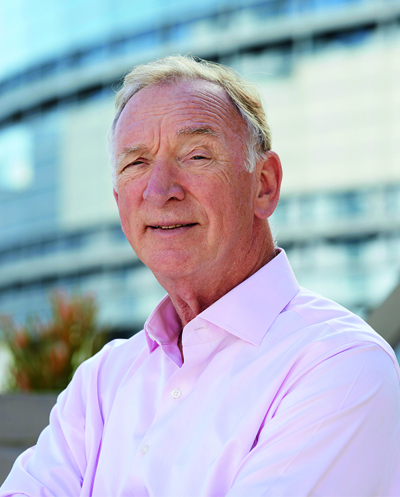
Dr. Richard Lewis is principal investigator of a stem cell trial at the ALS Clinic.
Until now, ALS has frustrated researchers with a notoriously impenetrable monolith. Only 5% to 10% of patients carry genes known to cause the disease. Without the ability to biopsy brain and spinal tissue, little is understood about its mechanisms. In the absence of biomarkers, physicians can only diagnose ALS after it has already taken hold, and the three Food and Drug Administration approved treatments do little to slow its progression. The highly specialized, resourceful clinicians at Cedars-Sinai’s ALS Clinic, an ALS Association Certified Treatment Center of Excellence, can only leverage tools and technologies to support their chief goal: to preserve quality of life as patients become paralyzed and die.
Buoyed by breakthroughs in the study of stem cells, Cedars-Sinai investigators are challenging assumptions and evolving their questions about ALS. Because fresh progress in the disease is fueled by the body’s cells at their most naive state, the ALS Clinic team has embarked on a new clinical trial to test the safety of stem cell implantation directly into the cerebral cortices of ALS patients. Scientists are growing cells from ALS patients in petri dishes to model the disease. Having built the largest library of hyper-specific disease data, the team is reconsidering whether ALS is not one disease but a collection of conditions. They aim to differentiate between genetic and sporadic forms of ALS, and scour the models for the earliest signs of cellular decline. Every approach takes square aim at the ultimate questions: Why do patients develop ALS, and how can we stop the suffering?
A STARRING ROLE FOR STEM CELLS
For nearly 20 years, Clive Svendsen, PhD, executive director of the Board of Governors Regenerative Medicine Institute and the Kerry and Simone Vickar Family Foundation Distinguished Chair in Regenerative Medicine, has cultivated a multipronged sneak attack against neurogenerative disease. The approach aims to replace diseased astrocytes, star-shaped cells that support motor neurons. In ALS, diseased astrocytes play a role in motor neuron death, which causes paralysis. Glial cell line-derived neurotrophic factor (GDNF) is a potent growth factor that can protect motor neurons, but delivery to patients is difficult since GDNF is too large to cross the blood-brain barrier. So, years ago, Dr. Svendsen generated a line of human neural progenitors that can become astrocytes, and genetically engineered them to release GDNF.
In 2007, he and colleagues at the University of Wisconsin published a paper in PLOS One demonstrating that, when implanted into the lumbar spinal cords of rat models of ALS, the neural progenitors became astrocytes and released GDNF. After Dr. Svendsen joined Cedars-Sinai, further success showing the function and safety of the cells in animal studies earned his group an $8 million grant from the California Institute for Regenerative Medicine (CIRM). The team also was granted FDA approval, in 2016, to begin the 18-patient trial in which Ashley Fisher participated.
“Proving the safety and getting the cells to survive was a huge lift,” Dr. Svendsen says. “Drug development can stop at any point, so to have 18 patients and no safety issues—we’re very excited to move forward.”
The Phase I/IIa study was overseen by Pablo Avalos, MD, associate director of Translational Medicine at the Board of Governors Regenerative Medicine Institute. A neurosurgical team, led by J. Patrick Johnson, MD, co-medical director of the Cedars-Sinai Spine Center and vice chair of the Department of Neurosurgery, injected the cells into the exact part of the spinal cord that controls movement in either the right or left leg. The procedure was found to be safe for all patients. Investigators also observed, as a secondary measure, that the cells slowed disease progression in the treated leg in some patients, though this did not reach overall statistical significance. ALS typically causes decline in both sides of the body at the same rate. Since patients received cells in only one side of the spinal cord, their own untreated leg acted as an “internal control” for comparison.
Postmortem spinal tissue revealed that the stem cells were still alive and producing GDNF in the treated side of the spine for up to three-and-a-half years after transplantation. Investigators continue to study postmortem spinal cord and brain tissue, searching for differences in dysfunction between lower motor neurons located in the spinal cord and upper motor neurons found in the motor cortex of the brain. While clinicians can detect ALS with electromyography and strength assessments that indicate lower motor neuron function, they have no measurable disease markers for upper motor neuron changes.
Frank Diaz, MD, PhD, a neuromuscular medicine specialist and assistant professor of Neurology, who diagnoses and treats patients in the ALS Clinic, is employing novel MRI techniques to identify markers of upper motor neuron dysfunction in postmortem brains and in living patients. He hopes to detect signal abnormalities in upper motor neurons. When paired with corresponding clinical data, this could support the development of more specific diagnostic tools, he says.
“Some patients do not have obvious clinical evidence of upper motor neuron dysfunction early on, which leads to delays in diagnosis and treatment, and even precludes their participation in clinical trials,” Dr. Diaz says. “Identifying markers of dysfunction will help us tremendously in understanding how the disease starts in the first place.”
IN HIS OWN WORDS: CLIVE SVENDSEN, PHD, ON THE PATIENT WHO INSPIRED HIS WORK
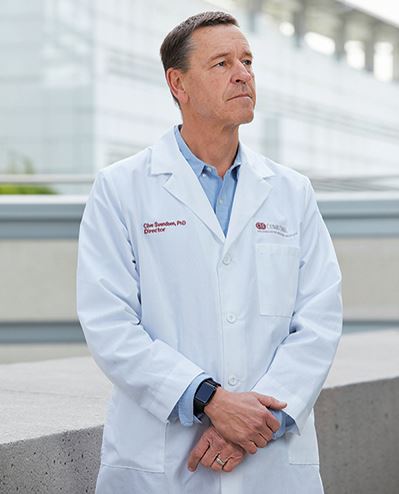
Clive Svendsen, PhD
“I had worked on Parkinson’s disease for years—but in 2002, in Wisconsin, I met Jeff Kaufman , a young patient with ALS. I was astounded at the horror of this disease—he had been an athletic man, a wonderful lawyer in his 30s with a lovely wife and four beautiful kids, when he was diagnosed. When I met him, he could only use his eyes to communicate through a computer. His first words to me were “Can stem cells help me?”
At the time, I was founder and co-director of the Stem Cell and Regenerative Medicine Center at the University of Wisconsin. After encountering Jeff, I dove into the literature and started asking questions. I realized that the approach we’d been studying in other neurodegenerative diseases—using astrocytes and GDNF as a protective strategy—could also protect motor neurons that die in ALS. So I switched my focus, applied for funding from the ALS Association and it’s all cumulated into the work we do now.
When I met Jeff, he had been fighting the disease for 10 years. He died at 54 in March 2010, and I’ve now been fighting for 20 years. While we don’t have the knockout blow yet, we have landed some punches, and I’m not going to stop.”
MODELING DISEASE ORIGINS
In tandem with clinical trials, Cedars-Sinai investigators are leveraging patient-derived stem cells to model the disease in the lab and in animals.
Ritchie Ho, PhD, assistant professor of Neurology and Biomedical Sciences, who runs a lab at the Cedars-Sinai Board of Governors Regenerative Medicine Institute, is investigating motor neurons made from induced pluripotent stem cells (iPSCs) of ALS patients to identify the earliest signatures of disease and elucidate how disease pathways differ among patients based on their genes.
Dr. Ho’s research trajectory syncs with the groundbreaking discovery, in 2006, of iPSC technology. His PhD thesis focused on the mechanisms of stem cell reprogramming: the complex process of turning blood or skin cells back into a blank slate, so they can be coaxed, by the addition of specific protein growth factors, to become any type of cell in the body.
In a 2021 paper published in Cell Systems , Dr. Ho uncovered molecular differences between iPSC models of patients with sporadic and familial ALS. Though sporadic and familial disease lead to the same outcome, for the 90% of ALS patients without an identified gene associated with the disease, there is no explanation for its development.
“The hope is that, for those patients, we can use their own stem cells to analyze their genetic background,” Dr. Ho says. “Maybe the cause of their disease is genetic—there could be an undiscovered constellation of gene mutations. Maybe it’s not just one gene that causes ALS, but a series of unfortunate events, a critical mass gone wrong.”
This leads to questions about whether investigators can stratify ALS and its pathological processes to segment the disease more specifically based on whether the cells experience problems clearing proteins, processing energy or conducting other activities.
“ALS is potentially an umbrella disease—we don’t know, out of 1,000 patients, if there could be 100 different groups,” Dr. Ho says. “We’re coming to the point where the spheres of medicine and basic research are at a conjunction. We solve these unknowns through integrating patients’ genetics with how their cells behave in the petri dish and which RNA and proteins they express. Then we can connect these data patterns back to what’s going on in patients’ bodies as observed in the ALS Clinic and eventually develop truly personalized medicine. We hope to predict how a person will develop ALS and treat their cells before they degenerate.”
BUILDING THE FUTURE OF ALS RESEARCH
At the Cedars-Sinai Biomanufacturing Center, Dr. Svendsen’s group, in collaboration with Dhruv Sareen, PhD, the center’s executive director, is building the largest-ever collection of iPSCs from ALS patients as part of the nationwide Answer ALS initiative. The stem cell lines are paired with an open-source repository of corresponding clinical, genetic, molecular and biochemical information, amounting to the most comprehensive collection of ALS data in history.
The project is groundbreaking in scale and specificity, and intended to encourage and enable researchers everywhere to leverage the data to develop deeper questions—and pursue answers. Earlier this year, Dr. Svendsen’s group and collaborators published a paper in Nature Neuroscience utilizing Answer ALS data to uncover disease subtypes.
Though stem cells have vastly expanded our insights into ALS, the grim reality of the disease and the lack of treatments are never far from mind for devoted investigators.
“This is how depressing it is—we started collecting the lines four years ago, and 700 of those 1,000 patients have died,” Dr. Svendsen says. “That’s why we’re determined to do these big projects to understand more. We’re just starting to crack the surface in uncovering clusters of patients with certain characteristics, and we’ve started breaking down questions about what causes it. You can’t design a drug treatment for a disease if you don’t know its cause.”
Challenges in finding a cause remain: No model of any disease is perfect. What might get lost or introduced into iPSCs during “reprogramming” to make them less reliable imitators of how the disease behaves in the body? Investigators hope to address this open question to improve the best tools we have.
STEM CELLS CONTINUE TO SHINE
Last year, with an additional $12 million from CIRM, Cedars-Sinai investigators launched another first-ever, 16-patient safety trial, transplanting the GDNF-producing stem cells into the brain, in a region of the motor cortex that controls hand movement. The research team hopes the operation will leave patients with nothing worse than a scar under their hairline, and that they’ll see a positive effect on hand use, which the team will monitor in the ALS Clinic.
Clinical trials proceed slowly, and participant selection is a frustrating paradox: Patients must show early stages of paralysis to qualify, by which time they likely cannot regain long-term function of their bodies. But investigators hope that the process of proving safety will ultimately lead to an efficacy trial combining delivery to both the spinal cord and motor cortex.
“This is a protective strategy and it’s definitely about timing,” Dr. Svendsen says. “If all the motor neurons are dead, no matter how much progenitor cells and GDNF we deliver, they’ve got nothing to act on. In the future, we can intervene earlier in the disease, and that’s when we might start slowing the progression.”
Cell-based regenerative medicine isn’t the only approach that offers hope for ALS patients. Scientists at Houston Methodist are focused on immunotherapy: A 2022 study published in Neurology proved the safety of the intravenous application of T cells meant to slow ALS progression. Another trial, completed at six sites including Cedars-Sinai, met safety benchmarks for the infusion of ALS patients’ own bone marrow stem cells into their spinal fluid; but a Phase III study, published in a 2022 Muscle Nerve paper, did not meet its efficacy endpoint.
Dr. Lewis remains focused on stem cells’ potential for progress, and hopes that continued clinical trials will validate the approach.
“We are not going to be able to convert this into a therapy for people who currently have the disease,” he says. “But the scientific question is an important one, and the fact that the stem cells survive is crucial—it’s a start.”
TRICKING TIME IN A DISEASE OF AGING
Descriptions of major disorders like cancer, lung disease and heart disease date to at least 1500 B.C.—but ALS wasn’t identified until 1869, and wasn’t widely known until 1939, when baseball player Lou Gehrig was diagnosed.
Because ALS typically presents between the ages of 55 and 75, perhaps a shift in life expectancy—from under 40 in premodern times to a turn of the 20th century increase—could explain why the neurodegenerative disease was undocumented before the modern era. But if ALS is a consequence of our expanded longevity, what explains why it strikes in old age? What are the hazards in aging?
After discovering, as outlined in a 2016 Nature Neuroscience study, that motor neurons derived from iPSCs of ALS patients more closely resemble fetal cells than adult cells, Ritchie Ho, PhD, is attempting to “age” them in the lab to more faithfully model late-onset disease. He hopes to accelerate iPSC maturation—to synthesize a lifespan—and search for signs of pre-programmed cellular “events” that instigate the onset of ALS.
“If we can activate the aging program, we can start to see the neurons become diseased and die, and how,” Dr. Ho says. “We’re trying to look at the very early origins of ALS, assuming there’s something in the neuron that sets it up to die, and trying to understand what that is in order to intervene before it manifests in paralysis.”
To try to trick time, Dr. Ho is measuring gene expressions of iPSC-built motor neurons against gene expressions in tissue from ALS patients and healthy patients. He’s attempting to adjust the manufactured cells to better mimic adult cells by adding small molecules known to switch genes on and off.
In theory, the artificial, expedited aging of iPSCs can help identify what triggers disease onset, Dr. Ho says. “If we can see the full cycle of ALS from start to finish—how the cellular-energy expenditure is different, how proteins accumulate and, at the last stage, how cells are dying—we can pick it apart and see where along a lifespan the disease processes happen.”