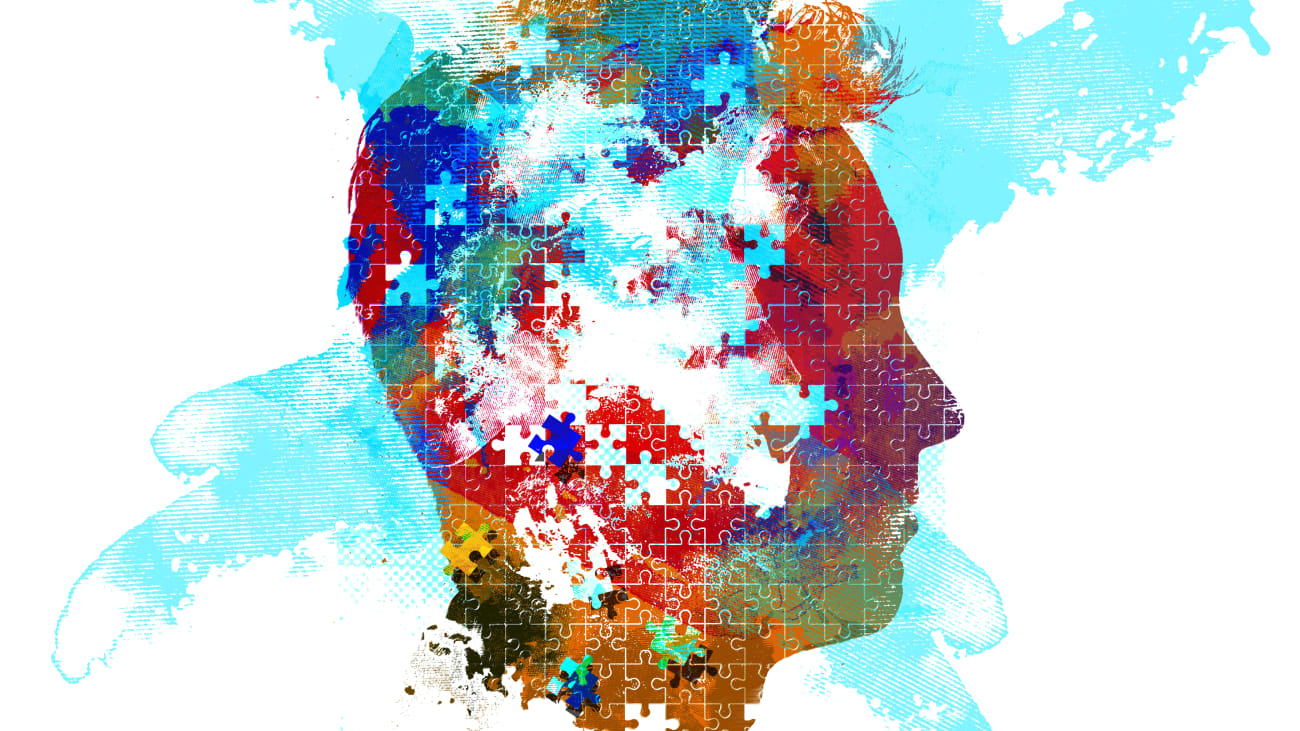
Cedars-Sinai is home to a level 4 epilepsy center—the highest level of accreditation from the National Association of Epilepsy Centers—and one of the few epilepsy monitoring units in the Los Angeles area. Here, experts can observe patients and their seizure activity in real time with the goal of reducing the frequency and burden of seizures in the future.
Patients in the epilepsy monitoring unit have electrodes implanted in their brain as part of their prescribed treatment so that physicians can determine where their seizures originate. These same electrodes also offer precious and rare opportunities for research into how the human brain functions and how it is affected by disease.
“The regions of the brain where the electrodes are implanted are important to understand epilepsy, as well as learning and memory,” said Ueli Rutishauser, PhD, professor of Neurosurgery, Neurology and Biomedical Sciences; director of the Center for Neural Science and Medicine; and the Board of Governors Chair in Neurosciences at Cedars-Sinai.
“The electrodes have microwires that allow us to observe the activity of individual nerve cells, which is key in the study of learning and memory. Much of that kind of work relies on animal studies, but human cognitive abilities are far more complex, so this clinical scenario provides an incredible opportunity to understand how human cognition works,” he explained.
Jeffrey Chung, MD, director of the Cedars-Sinai Epilepsy Program and a neurologist who treats patients within the unit, notes that many of the unit’s patients are happy to volunteer for the additional research efforts.
“Those of us who treat patients with epilepsy know they often experience memory deficit over time,” said Chung. “While the research they participate in will likely not benefit them directly, it may lead to methods that improve memory or prevent its loss for others in the future.”
Recent major progress using this approach has focused on performance monitoring and the formation of memories.
Performance Monitoring: An Internal Critic
Performance monitoring, an executive function that allows us to observe and critique our own behavior, is critical to understanding how we can regulate our behavior. Researchers at Cedars-Sinai are particularly interested in studying error monitoring, which is an important aspect of performance monitoring.
“An example would be calling someone by the wrong name or hitting ‘send’ on an email too soon,” said Rutishauser. “Right after you do it, you get a strong sense that you made a mistake. No one has to tell you; it’s an internally generated signal.”
The team is interested in the study of error monitoring because impairment of this process can have severe consequences. One main theory behind obsessive-compulsive disorder is that it may be due to an overactive error monitoring process, while schizophrenia may be in part due to an underactive error monitoring process.
To better understand how error monitoring works, the team studies the medial frontal cortex of patients—the area of the brain thought to detect errors—using electrodes already implanted in that area of the brain for clinical seizure observation.
Patients are asked to complete tasks on a computer that is constructed to induce errors. For example, one task displays the word for a color, but the word itself is displayed in a different color. In this task, the word “red” may be displayed in green ink. The patient is asked to name the color of the ink, not the word itself. This design creates conflict; many patients choose red as the answer and quickly realize their mistake.
“When you watch activity in this part of the brain, it’s astonishing that relatively little activity is present while a decision is being made,” said Rutishauser. “But after you make the decision, that part of the brain becomes very active. Therefore, we hypothesize that this area of the brain evaluates the consequences of decisions.”
A novel finding from this study was that error monitoring is a domain general process, meaning this area of the brain can monitor behavior in different tasks, even if we have never done them before. Previously, each part of the brain was thought to be specialized for different functions, but that is not the case.
“Once we identified how neurons signal when we made an error in one task, we also knew how they would signal an error in another task,” said Rutishauser.
Adam Mamelak, MD, professor of Neurosurgery and director of the Functional Neurosurgery Program at Cedars-Sinai, performs the procedure to implant the electrodes that make this work possible. He believes this research has helped illuminate a clearer, bigger picture of brain function.
“What this work demonstrates is how brain regions work as a network rather than as individual nerve cells,” said Mamelak. “It’s not a single nerve cell that identifies an error signal. There is a whole network of nerve cells activating in patterns that look complex but actually align in a meaningful way. These studies characterize a pattern of a collection of neurons at a given time to say that specific behavior is representative of a meaningful function.”
Episodic Memory: A Pathway to Treat Memory Disorders
This team used the same approach in a second study that explored memory. This time, activity was recorded from the medial temporal lobe—the area of the brain known to be important when forming and retrieving memories.
Although life happens continuously, memories are thought to be stored in chunks called episodes. The major question at hand is what actually defines the beginning and end of those episodes. If the brain does not define this properly, episodes fuse together, resulting in segmentation deficits, such as those observed in certain types of dementia.
“Proper segmentation of episodes is what triggers the formation of new memories,” said Rutishauser. “If no new episode starts, no new memories are made.”
The team tested the idea that a new episode starts when there is a cognitive boundary, or a break in the narrative. For example, if you are having a conversation with a friend and get a phone call from your boss in the middle of it, that would create a cognitive boundary.
“We reasoned that the memory system of the human brain would have specialized cells that detect cognitive boundaries,” said Rutishauser.
Using the same type of electrode, the research team monitored brain activity as patients watched movie clips they had never seen before and attempted to recall the scenes later.
“There were three types of movie clips,” said Rutishauser. “Some consisted of one continuous scene with no boundaries. Some had soft boundaries, like seeing one group of people in an office and then watching the same group going out to lunch, and some had hard boundaries—cutting to drastically different scenes.”
Through observation, investigators saw some neurons increased activity after both hard and soft boundaries. These were labeled “boundary cells.” Other neurons increased activity only after hard boundaries and were labeled “event cells.” Rutishauser compares storing new memories to creating folders on a computer. When boundaries are presented, one folder is closed and a new one is opened.
“When it is time to assess whether we have seen something before or not, the memory system compares the pattern of activity evoked by the to-be-assessed stimulus to previous patterns of activity stored in memory,” said Rutishauser. “If it finds one that is similar, it opens that ‘folder’ to access those memories.”
The researchers theorized that the peaks in brain activity after cognitive boundaries prime the brain to make a new memory. This theory was evaluated using two memory tests.
Participants first were shown still images and asked if they had seen them in the movie clips they had previously watched. Images were most likely to be remembered if they closely followed a cognitive boundary in the clip.
Next, participants viewed pairs of images from the movie clips and were asked which had appeared first. Participants had difficulty remembering the correct order of images that appeared on opposite sides of a boundary, an effect that would be expected if the brain had segmented those images into separate memory folders.
Rutishauser and his team also noted that when event cells fired in time with one of the brain’s internal rhythms, the theta rhythm—a repetitive pattern of activity linked to learning, memory and navigation—subjects were better able to remember the order of images they had seen. This is an important new insight because it shows that deep brain stimulation that adjusts theta rhythms could prove therapeutic for memory disorders.
These findings reveal a neural substrate for event segmentation and thereby provide important context for how to potentially restore or improve function of this system in cases where it is deficient. In the future, researchers hope to compare responses between people who have memory deficits and those who do not to determine whether cognitive boundary cells function differently in someone who has memory deficits.
“You have to understand how all cells in the brain are interacting at a network level before you can modulate it,” said Mamelak. “Understanding how the brain decides to keep or dismiss information is fundamental. It’s not hard to imagine the impact this research could have on developing therapies related to learning, concentration, memory and error monitoring.”