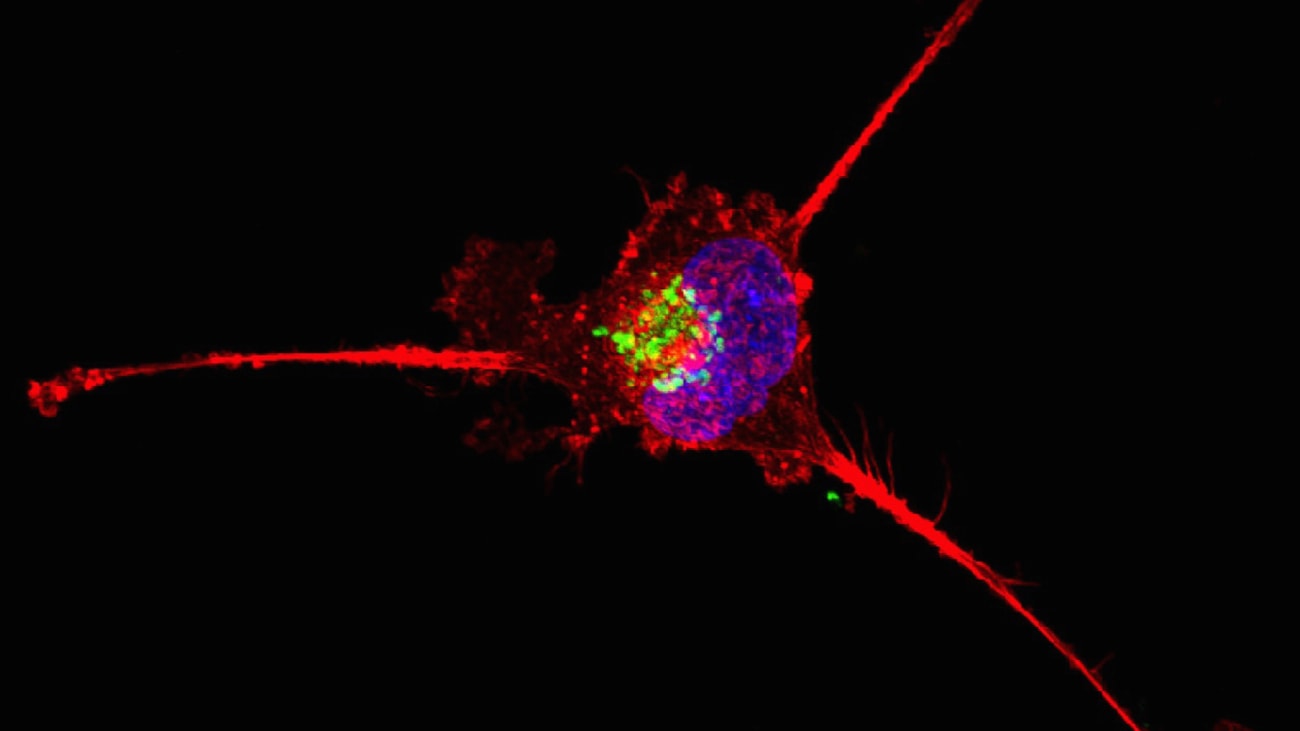
Triple-negative breast cancer (TNBC) is one of the field’s most challenging cancers. It doesn’t respond to available treatments. It often metastasizes into the brain, making it a challenge to reach with traditional anti-cancer drugs, as the blood-brain barrier (BBB) keeps many such substances out.
Now, after more than two decades of protein-design work and years of collaboration with pharmaceutical researchers and computer-simulation experts, Cedars-Sinai Cancer scientists are several steps closer to addressing this challenge. As is often the case, the solution to this big problem comes in a very small package: a nanoparticle.
The protein—designed and manufactured by Lali Medina-Kauwe, PhD, associate director of Basic Science Research at Cedars-Sinai Cancer—has been in the works since 2000. She had been developing it for HER2+ breast cancer when questions from colleagues prompted her to find out whether it could cross the BBB.
“I had never looked above the neck, because it’s breast cancer!” Medina-Kauwe said. “When I found my nanoparticle in the brain, I didn’t believe it at first. I tested it several ways, though, and in each case, it kept showing up there.”
She worked to optimize the nanoparticle’s BBB-crossing feature and tumor-targeting potential via both rodent trials and human induced pluripotent stem cells with BBB-on-a-chip technology pioneered at the Cedars-Sinai Board of Governors Regenerative Medicine Institute.
The approach enabled rapid progress in verifying the nanoparticle’s ability to cross into the brain and, even better, spurred a host of ideas about how best to use this feature to target brain-metastatic cancers, such as TNBC.
Optimizing the Nanoparticle for BBB Crossing
Rather than continue to spin her wheels in her lab, she asked a computer scientist colleague to develop a 3D simulation of the protein and how it moved and interacted with other cells and even atoms in the cellular environment. The collaboration was a game changer.
“I have been working with my ‘Frankenstein’ protein for more than 20 years, and I knew everything it could do—its size, functions and activities,” said Medina-Kauwe. “But I never got a picture of it until that collaboration, and it changed everything.”
Ravi Abrol, PhD, a computational modeling expert and associate professor of Biochemistry at California State University, Northridge, used high-powered computer simulations to open a world of “what-ifs” to Medina-Kauwe.
Instead of making the modifications in the lab first, new computer simulations allowed her to see the effects of potential nanoparticle design tweaks or even environmental variations, such as introducing a few ions here or there near the molecule, changing the pH of the environment, or exposing it to a peptide.
“It helped me streamline the protein design to better penetrate cells and to be more conducive to production at a higher yield, with cleaner activity and purer output,” Medina-Kauwe said. “I could have made a million modifications in the lab, but seeing the simulations helped me optimize the potential designs by modeling their movements and predicting the resulting activities much faster and more affordably.”
The latest rendition is “superior in every way to the original construct,” she said, and has shortened her nanoparticle’s expected journey to the clinic.
Crossing the BBB Into the Clinic
After making great strides with protein optimization and the mechanics of BBB crossing, Medina-Kauwe was still in need of a critical piece of the TNBC treatment puzzle: a drug to kill the tumors once the particle reached the cancerous cells. Enter James Turkson, PhD, associate director of diversity, inclusion and strategy at Cedars-Sinai Cancer.
“My lab develops tumor-toxic compounds that target cancer-specific pathways,” said Turkson, a pharmacology expert with numerous collaborations underway at Cedars-Sinai. “We’ve already shown that a particular compound we made causes TNBC models to shrink, and this is just one option we are exploring for insertion into Dr. Medina-Kauwe’s nanoparticle to fight tumors in the brain.”
The team’s initial collaboration showed therapeutic efficacy in mouse models of brain-localized TNBC after systemic administration. They are now working to optimize the drug and its delivery, as the compound is cleared quickly from the blood once given via intravenous injection. They expect they can strengthen the compound’s properties in the coming year or two to the point that it will be ready for initial clinical trials.
In the meantime, the team is advancing other promising drug candidates—some of which will also be carried by Medina-Kauwe’s nanoparticle—to target brain tumors and difficult-to-treat tumors elsewhere in the body. They are also beginning talks with clinical trialists and regulatory consultants to ensure they are prepared to advance the molecules to the clinic when the time is right. At each stage, the collaboration is constant and the progress is tangible.
“To make valuable compounds, you have to understand the biological entities you’re making and targeting, which requires an understanding of chemistry, mathematics, physiology, cell biology and—as we have seen—computer modeling to better grasp the molecules and their physical properties,” said Turkson.
One of his latest discoveries involves a naturally derived anti-cancer compound found in tea, which has demonstrated tremendous early potential against cancer cells.
“We are using convergent science principles throughout our work at Cedars-Sinai,” said Dan Theodorescu, MD, PhD, director of Cedars-Sinai Cancer and PHASE ONE Foundation Distinguished Chair and Director at the Samuel Oschin Comprehensive Cancer Institute at Cedars-Sinai. “Our work is anchored in the premise that combining physical, mathematical and biological sciences across the entire institution will allow us to solve difficult cancer problems and accelerate solutions for patients.”
References
- Alonso-Valenteen F, Pacheco S, Srinivas D, et al. HER3-targeted protein chimera forms endosomolytic capsomeres and self-assembles into stealth nucleocapsids for systemic tumor homing of RNA interference in vivo. Nucleic Acids Res. 2019 Dec 2;47(21):11020-11043. doi: 10.1093/nar/gkz900.
- Alonso-Valenteen F, Sances S, HongQiang W, et al. Systemic HER3 ligand-mimicking bioparticles cross the blood–brain barrier reducing intracranial triple-negative breast cancer growth. Research Square pre-print. https://doi.org/10.21203/rs.3.rs-1139650/v1.
- Yue P, Lopez-Tapia F, Zhu Y, et al. Novel azetidine-based STAT3 inhibitors induce misfolded protein response, endoplasmic reticulum stress and mitophagy, and inhibit breast tumor growth in vivo [abstract]. Proceedings of the American Association for Cancer Research Annual Meeting 2022; 2022 Apr 8-13. Philadelphia (PA): AACR; Cancer Res 2022;82(12_Suppl):Abstract nr 5476.
- Zhu Y, Yue P, Dickinson CF, Yang JK, Datanagan K, Zhai N, Zhang Y, Miklossy G, Lopez-Tapia F, Tius MA, Turkson J. Natural product preferentially targets redox and metabolic adaptations and aberrantly active STAT3 to inhibit breast tumor growth in vivo. Cell Death Dis. 2022 Dec 6;13(12):1022. doi: 10.1038/s41419-022-05477-2.